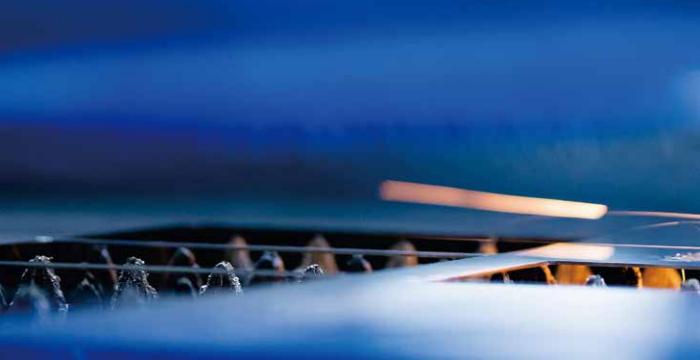
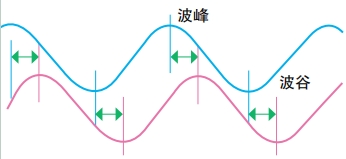
What do a DVD player, supermarket scanner, printer, fiber optic internet connection, and industrial metal cutter have in common? They all work thanks to lasers. DVD players and supermarket scanners use lasers to read data. Laser printers and fiber optic cables use lasers to transfer data from one place to another. And an industrial metal cutter uses lasers to vaporize metal. In many types of surgery, lasers carefully cut or vaporize skin or tissue.
All lasers may be hazardous to the skin or eyes if used incorrectly. But they are also incredibly common and useful. “I never leave home without lasers,” says astrophysicist Neil deGrasse Tyson. He regularly uses a green laser pointer to indicate sights in the night sky.
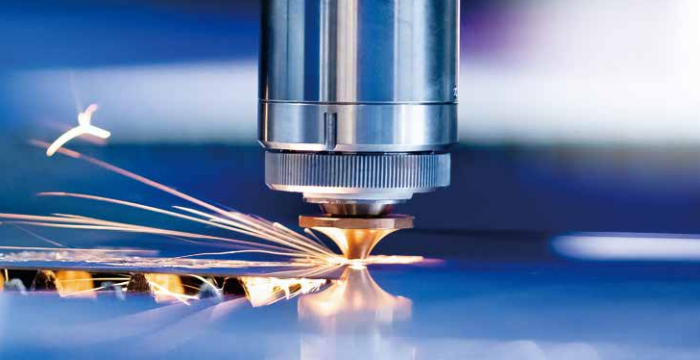
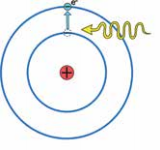
The word laser stands for “light amplification by stimulated emission of radiation.” That mouthful of terms describes how a laser works. Basically, it uses light to generate even more light. Lasers are not like flashlights or other typical light sources. They have three very important properties. Laser light is monochromatic, collimated, and coherent.
Monochromatic means all one color. A laser emits a single frequency only. Collimated means brought into line. The light from a laser all goes the same direction and forms an extremely narrow beam that focuses a lot of energy onto a very small area. Coherent means unified. Light is coherent if all its photons have the same frequency and the phase difference is constant, or the same at any time. Usually, though, light coming from a very small point will be very weak and dim. Lasers are exciting because they are coherent as well as very powerful and bright.
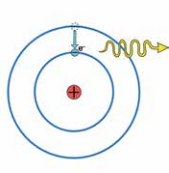
一个光子
How a Laser Works
Excited Electrons
Lasers don’t happen naturally. Physicists and engineers figured out how to make these narrow, powerful beams of light. A laser works because of a special interaction between atoms and photons of light.
This simple model of an atom shows a nucleus surrounded by electrons traveling in different orbits. This model is not realistic–the electrons actually form more of a blurry cloud. However, the important part of the model is that each orbit represents a different energy level. “Think of it like rungs of a ladder or floors in a building,” says Tyson.
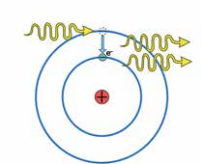
If a photon hits an electron at its normal or ground orbit, and that photon happens to have the exact amount of energy the electron needs to jump to the next orbit, the electron then will absorb the photon and jump to a higher orbit. The electron is said to be in an excited state. Almost immediately, though, the electron will get “homesick” and jump back down. As it jumps back, it will emit a photon that’s exactly the same as the one it absorbed. This is called the “spontaneous emission.”
Just like someone getting bored at a party, it doesn’t stay excited for very long.
Now think of an electron that is already in an excited state. The electron is sitting on the energy ladder at a level above its usual rung, and for the time being not very “homesick” (the case will be explained in the next section) . If we then send out a photon to hit it, again with the correct amount of energy – the energy the electron had needed to move up to its current place – guess what is going to happen?
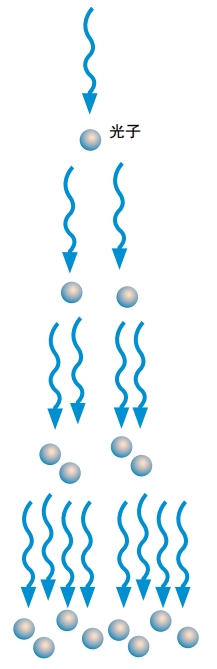
This is where interesting thing happens: the electron, on being hit with that photon, emits another identical photon, and moves down to its ground orbit! That’s what the electron does and this process is called the “stimulated emission.”
By sending a photon to hit the excited electron, we stimulated it to emit out an identical photon, and return to the ground state. This is the stimulated emission of radiation part of the word “laser.”
Now think of many identical electrons, all in the excited state. We send out just one photon (with the correct amount of energy) to hit one of those electrons. The one that is hit emits out an identical photon. Do you see how things are going to turn out? This emitted photon, and the original photon we used to hit the electron – two photons now – will go on to hit two more excited electrons, and cause them to each emit an identical photon. So one photon becomes two, then four, then eight, at an exponential rate. And all of them are identical. This is the light amplification part of the word “laser.”
Photons are the basic components of light. Such a large amount of identical photons, when put together, can form a strong, powerful beam of light – and that is laser beam.
Population Inversion
How can we make electrons stay in excited states and not wanting to move down? In a typical substance, there will always be more electrons in their ground states than in excited states. This is called thermal equilibrium. However, pumping lots of energy into some types of materials will cause more excited states than ground states. This is called population inversion.
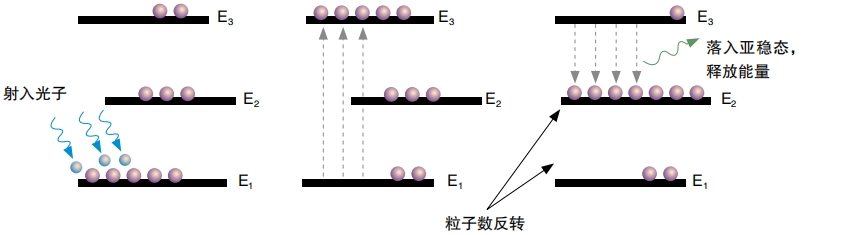
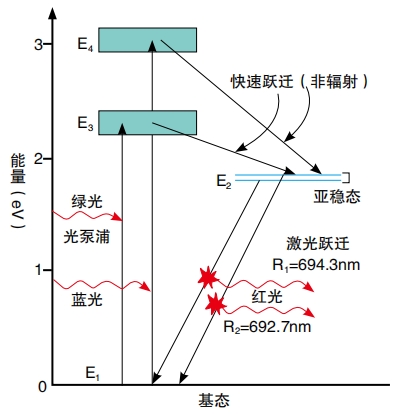
For population inversion to remain stable in a material, the electrons in its atoms must actually jump up more than one level into a highly excited state, also called a pump state, before dropping down to a less excited state, called a metastable state, and eventually back to a ground state. A metastable state has that name because electrons will stay there a bit longer than they will stay in the higher energy pump state. In materials with metastable states, it is possible for more electrons to end up in excited rather than ground states. These materials make the best lasing mediums.
The first lasing medium ever used was the gem ruby. However, lots of materials, including gases, liquid dyes, and solids, also work as lasing mediums. The difference in energy between the metastable state and ground state of a material determines what types of photons will interact with its electrons. This determines the frequency, or color, of the laser light produced.
The Optical Cavity
Achieving population inversion and getting lots of electrons into excited or highly excited states isn’t enough to make a laser. There also need to be enough photons flying around to hit those excited electrons. To accomplish this, lasers enclose the lasing medium in a space called the optical cavity. It is usually cylindrical, or rod shaped. Inside this cavity, on either side of the lasing medium, two mirrors face each other. The mirrors bounce light back and forth through the material. On each trip through, photons are likely to interact with excited electrons and release even more photons. Only the photons in the light path in the back-and-forth reflection direction between the plane mirrors are amplified, and there is no light amplification effect in other directions.
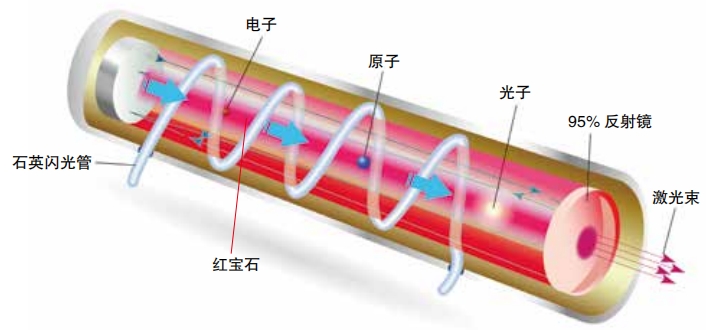
In a simple laser, one of the two mirrors is designed to let a small amount of light through. This light that escapes forms the laser beam.
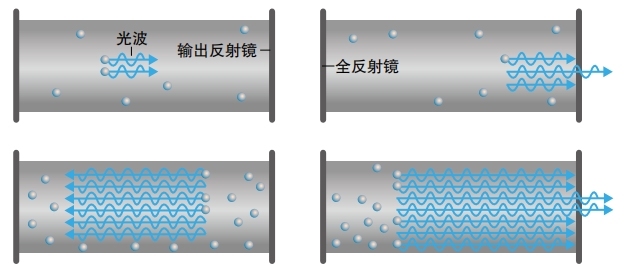
Pumping a Laser
It takes energy from the outside to boost electrons into excited or highly excited states to achieve population inversion. This is called pumping. In most lasers, this outside energy comes from lamps, electricity, or another laser. The very first lasers were pumped with flash lamps. These light sources are often used in photography because they provide a sudden burst of very bright light.
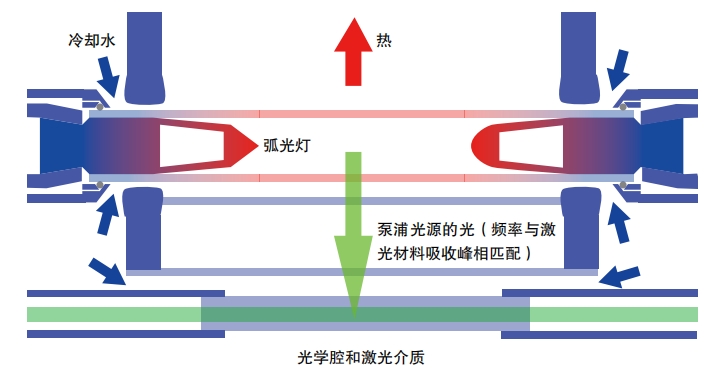
When an electron transits from the ground state to the excited state, it needs photons to give it just the right energy to absorb. The energy a photon has is given by the equation E=hv, where v is the frequency of light, and his the Planck constant. We need a sufficient proportion of the light emitted by the pump with a frequency of v.
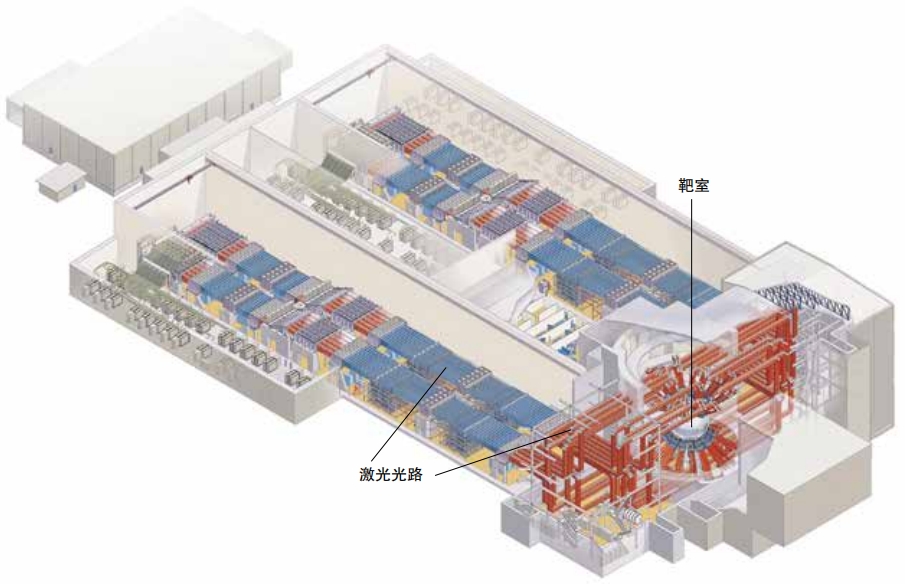
The pumping energy has to get to the lasing medium inside the optical cavity. One way to do this is to place a rod-shaped energy source along the side of the rod-shaped optical cavity. Another method is to wrap an energy source around the optical cavity in a spiral.
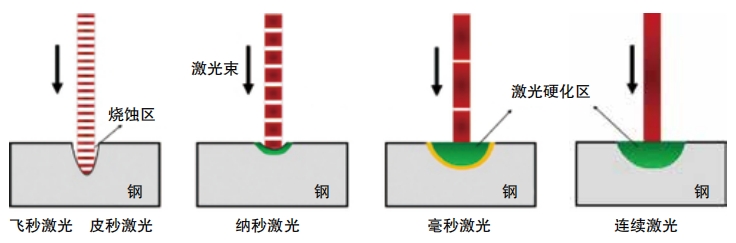
When you turn on a laser, its energy source begins pumping. This excites atoms (the electron jumps to a higher energy level) in the lasing material until population inversion happens. Then, as electrons drop down to lower energy states during stimulated emission, they emit photons. Some of these photons will be going in the wrong directions. But some will head straight for one of the mirrors. These will bounce back and forth, duplicating themselves every time they interact with excited atoms. The mirror setup maintains the directionality of the light. Very quickly, the number of identical photons will multiply. The system is now lasing!
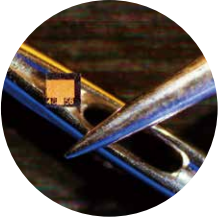
Some laser emits a continuous, uninterrupted beam of light, while others operate in pulses. One of the main differences between them is the power output. A laser’s power is equal to the amount of energy delivered per unit of time. A continuous six- thousand-watt laser always releases six thousand watts of power. Either a laser pointer or the one used for cutting or welding is a continuous laser. Pulsed lasers produce peak power. If you pump the same amount of energy into a laser but then keep shortening the amount of time it takes for that energy to release, the amount of power delivered per pulse will keep increasing. A one hundred watt pulsed laser can release ten thousand watts in each pulse! “For me, laser light is the most beautiful light in the world,” says Ursula Keller. She is a physicist whose 1991 invention made femtosecond lasers possible for the first time.
Varying the Laser Beam
Amplification
Passing an initial laser pulse through more optical cavities containing additional lasing mediums can further amplify the light. This increases the power of the laser pulse. The most powerful lasers in the world contain complex systems of mirrors, glass, and optical cavities. Some take up entire buildings!
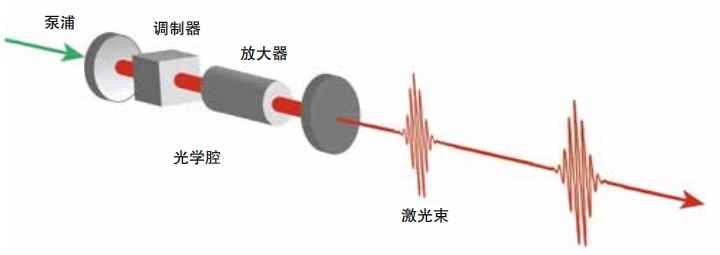
Tuning
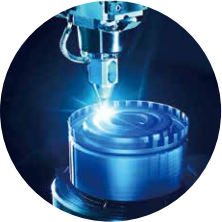
Tuning a laser changes the color of light that it emits. Remember that a laser beam only contains a single wavelength. Some lasing materials, though, have the potential to emit several different wavelengths. The setup of mirrors and other optical elements inside the optical cavity determines which wavelengths bounce back and forth and amplify inside the cavity. If this setup changes, then a different wavelength will get amplified.
Modulation
Engineers may add modulation to continuous or pulsed lasers to make it possible to control various aspects of the laser beam. Modulating a laser beam simply means changing it. Modulation may dim or pulse the beam or change aspects of the light, such as its polarization.
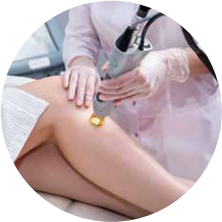
This is especially important in fiber optic communications. A modulator turns electronic data from a computer into modifications to a beam of laser light. In the simplest case, 1s and 0s become on and off flashes of light. These travel at the speed of light down a fiber optic cable. At the other end, communications equipment translates these flashes back into electronic data.
How Are Lasers Used
Solid State Lasers
The first laser demonstrated used ruby, a solid material, as its lasing medium. One of the most common solid materials used today is the crystal Nd-YAG (neodymium-doped yttrium aluminum garnet). It is usually pumped with a tube-shaped flash bulb or laser diodes and can be continuous or pulsed. It typically emits infrared light. Cosmetic doctors often use this type of laser to remove hair, tattoos, and skin blemishes. In manufacturing, this type of laser can engrave, etch, or mark a variety of metals and plastics. Nd-YAG lasers can also be used to cut or weld steel. The laser light cuts material in a very fine, controlled manner.
Melt and blow or fusion cutting uses high-pressure gas to blow molten material from the cutting area. First the material is heated to melting point. Then a gas jet blows the molten material out of the kerf, or cut area. Materials cut with this process are usually metals.
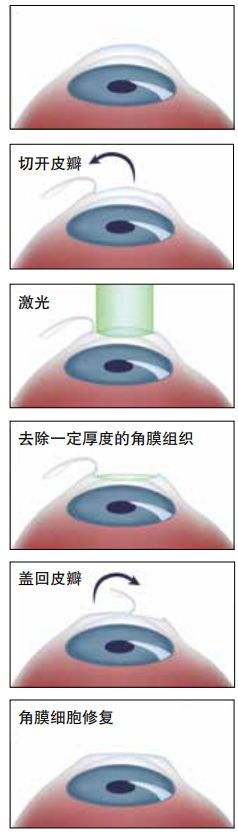
Gas Lasers
The most common gas lasers use a mixture of helium and neon as the lasing medium. They produce visible red light. Gas lasers are usually pumped with an electric discharge. An anode and cathode at each end of the gas reservoir pass the discharge through the gas. This causes a glowing plasma to form. This same process also makes a plasma-screen TV or a fluorescent lamp glow. He-Ne lasers are relatively cheap to make and easy to operate. So they are commonly used in science demonstrations. In the past, these lasers were used much more widely, in barcode scanners, CD players, microscopes, and more. Today, these products use smaller and more efficient laser diodes instead.
Excimer Lasers
An excimer laser combines a noble gas, either argon, krypton, or xenon, with a reactive gas, either fluorine or chlorine. With electrical pumping and high pressure, this lasing medium can produce laser light with a UV wavelength. These lasers are important in medicine because they don’t burn or cut material. Instead, they vaporize it. So they can remove very small areas of material without damaging any surrounding material. This is extremely useful in delicate surgeries, including LASIK eye surgery.
LASIK eye surgery fixes nearsightedness or farsightedness by making tiny changes to the curving shape of the cornea. The cells making up the cornea vaporize, creating a tiny plume of smoke. In this way, very small amounts of tissue can be removed from the cornea, changing its shape. The surrounding tissue is not damaged.
Excimer lasers are also an important part of the manufacturing process for making the very tiny circuits that power small electronic devices.
Semiconductor Lasers
The most common type of laser made today is also the smallest: the laser diode. This is an electrical component smaller than a penny. Electrical current pumps a semiconducting material inside the diode, which converts the electrical energy into laser light.
These lasers are an important part of many electronic devices, including DVD and Blu-ray players, e-readers, laser pointers, barcode scanners, and more. You likely use laser diodes every day without even knowing it!